Bioinspired Chemistry and Surface Models for the Activation of Small Molecules
Coordination Chemistry, Catalysis, Small Molecule Activation
Small molecules are constituents of the air we breathe (e.g. N2, O2, CO2) and take part in element cycles and various metabolic processes. Further representatives (e.g. H2, CO, CH4) are produced/converted in nature under anaerobic conditions or in industrial processes on large scales. Hence, small molecules such as those mentioned are readily accessible and comparatively inexpensive, so that it is highly desirable to include them into syntheses of value-added products or to use them as reservoirs of chemical energy (fuels). However, at the same time they are typically quite thermodynamically or kinetically stable, so that their utilization requires an activation step involving a metal complex entity. Work in our group concerns various aspects of small molecule activation, with the focus on atomic-scale insights and rational design of function using the methods of molecular chemistry. This includes the preparation of model compounds, ligand development, reactivity studies, an arsenal of spectroscopic methods and theoretical studies.
- Dioxygen activation at transition metal centers
- Bioinspired activation of N2, CO, CO2 and H2 at reduced metal centers or through metal cooperation
- Molecular Aluminosilicates as models, catalysts and precursors
- Metallophilic interactions involving bismuth
The catalysis of oxidation reactions is of major interest in various different fields, ranging from industrial and academic laboratories to biology. Enzymes realize the oxidation of hydrocarbons with high selectivities and efficiencies under physiological conditions. Hence, modern oxidation catalysis research uses such enzymes as an inspiration to create synthetic low-molecular-weight analogues, which are hoped to exhibit comparable activities. On the other hand a molecular oxidation chemistry can also receive inspiration from the active sites on the surfaces of heterogeneous oxidation catalysts aiming at a transfer to the homogeneous liquid phase and thus low temperatures. In turn, molecular chemistry can contribute to these areas by improving the understanding of elementary steps and electronic structure on the molecular level with the aid of model compounds. Such model compounds allow to create an image of the structure or reaction behaviour of the occurring key species, that might be selected structural motifs of oxidic solids, employed as heterogeneous catalysts, or the active sites of oxo transferring enzymes.
The Limberg group indeed uses both, the active sites of enzymes and solid materials, as paragons but also as objects of investigation to deal within one of its major research areas with metal-oxygen systems, that is, with O2 activation, with molecular metaloxo and dioxygen complexes as well as with the utilization of such compounds for the oxidation of hydrocarbons. In this context also mechanistic questions are intensely pursued.
This may be exemplified for the two different subareas as shown below:
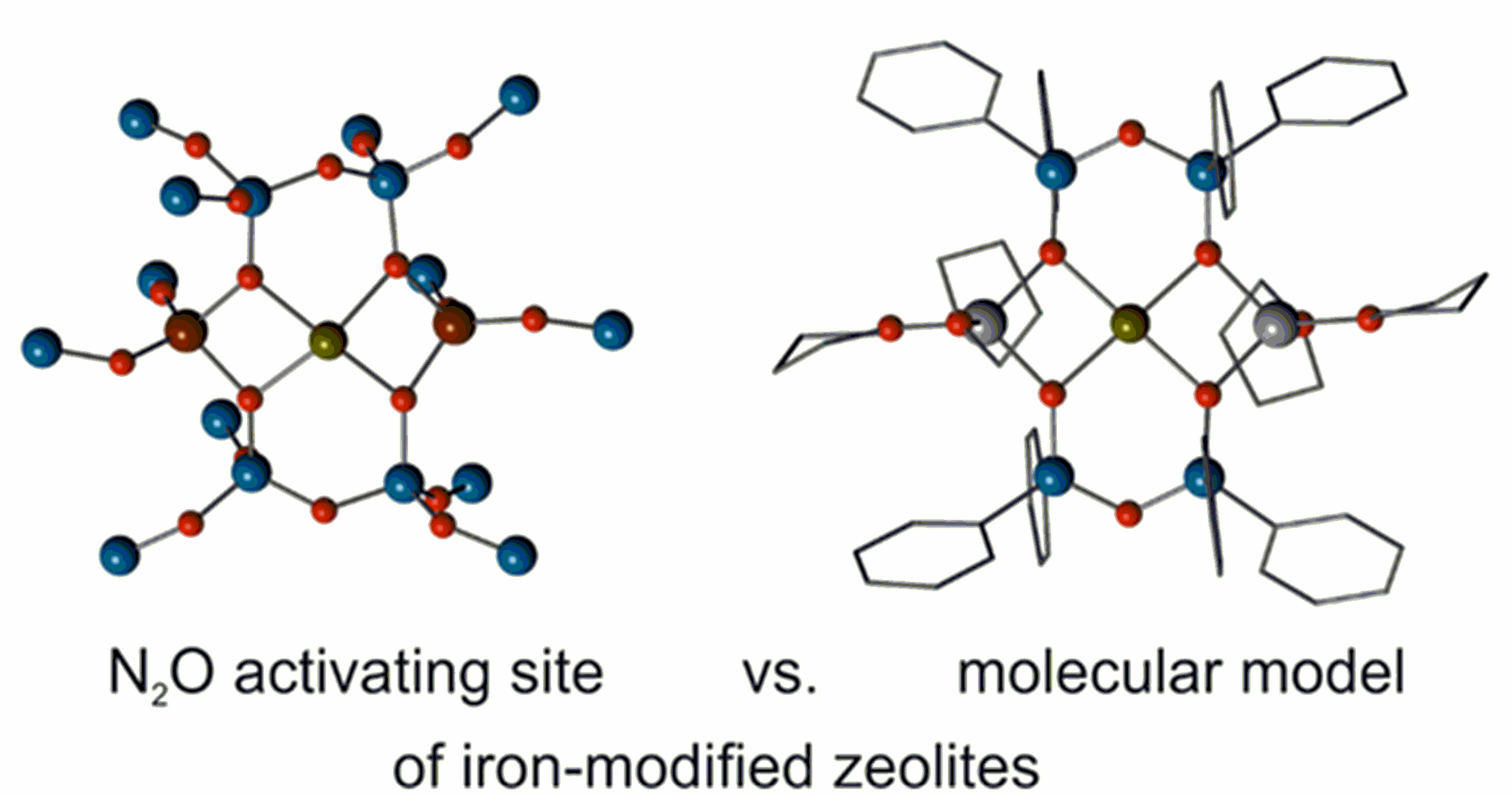
Despite many decades of research aiming at a molecular understanding of the mechanistic principles and reaction steps of catalytic oxidations on surfaces, still many questions remain unclear and many systems have be regarded as ill-defined. This inter alia is based on the problems one encounters during the characterization of intermediates and these problems have their origin in the heterogeneity of the surfaces featuring various species with different activities, which are averaged during the analytical investigation.
Therefore we are pursuing the functional modeling of individual structural motifs potentially occurring in heterogeneous catalytic processes by means of well-defined molecules, on the one hand to improve the understanding of such sites, on the other hand to create novel, functional molecules. In many catalyst the active component is supported on silica, which may be regarded as a large ligand. For the design of surface mimics therefore siloxide ligands are developed and employed. On the right the cut-out active site calculated for an iron-modified zeolite is compared with one of our model systems.
However, as mentioned above, not only surfaces are serving as paragons for the Limberg group but also enzymes which is the theme of the second subarea of O2 activation:
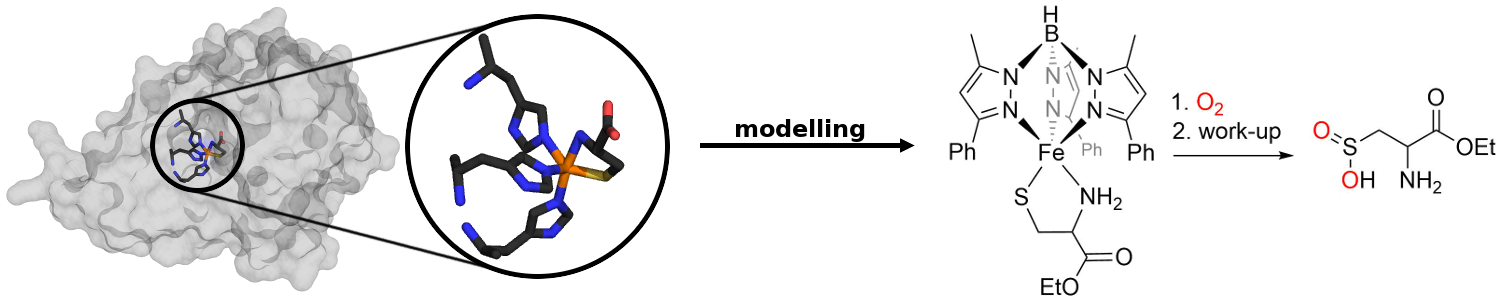
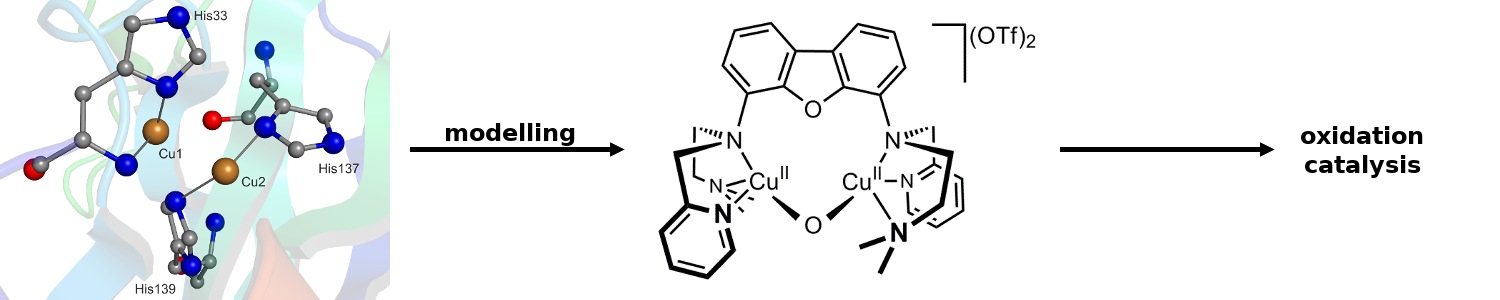
From nature various dinuclear metalloenzymes are known which are capable of activating dioxygen for the oxidation of hydrocarbons. The Limberg group is therefore developing dinuclear iron and copper complexes, which can be tested then with respect to their potential as functional models for the mentioned enzymes. Moreover, also the mimicking and understanding of mononuclear oxygenating proteins, like non-heme dioxygenases are targets of the groups research (see right).
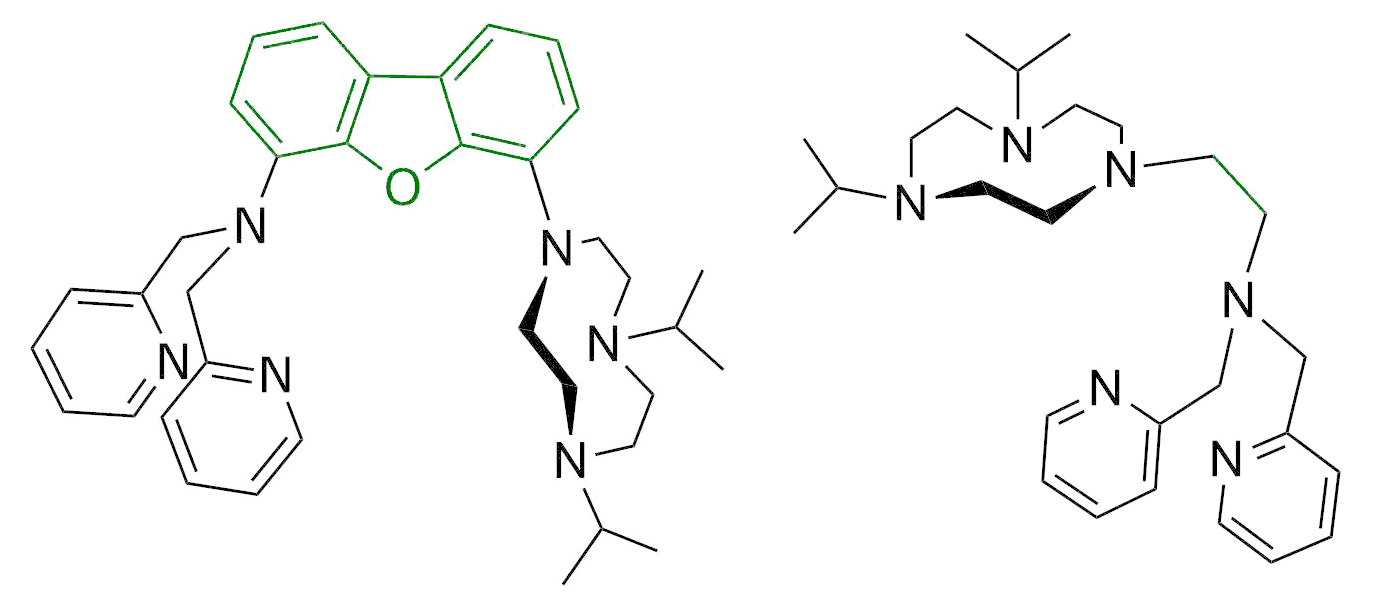
Enzymes are fascinating and inspiring catalysts also beyond the field of O2-activation. They allow for numerous interesting reactions in biological systems setting out from small molecules, like, for instance, N2, H2 and CO2. As in case of O2 the efficient utilization of such molecules requires a prior activation, and – again – enzymes employ metal centers for that, which leads to the Limberg groups second focal point dealing with the activation of small substrates like N2, CO2 and H2 with the aid of reduced or Lewis acidic metal complexes.
To reach the activation of small substrates on the one hand side complexes are synthesized where two metal centers (Zn, Fe, Mn, Cu, Mg, Ni) are fixed in a certain distance to each other via a special ligand matrix (see right), so that a chemical or electronic cooperation becomes possible.
Subsequently, the catalytic activities of these complexes are investigated.
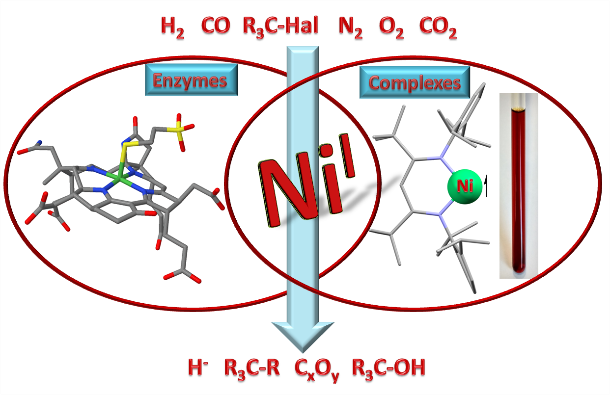
On the other hand low-valent complex metal fragments (e.g. with nickel(I), see right) are employed, which are capable of binding and activating small molecules reductively. Here too, often bimetallic moieties, which may arrange without a support by the ligand system, are playing a decisive role.
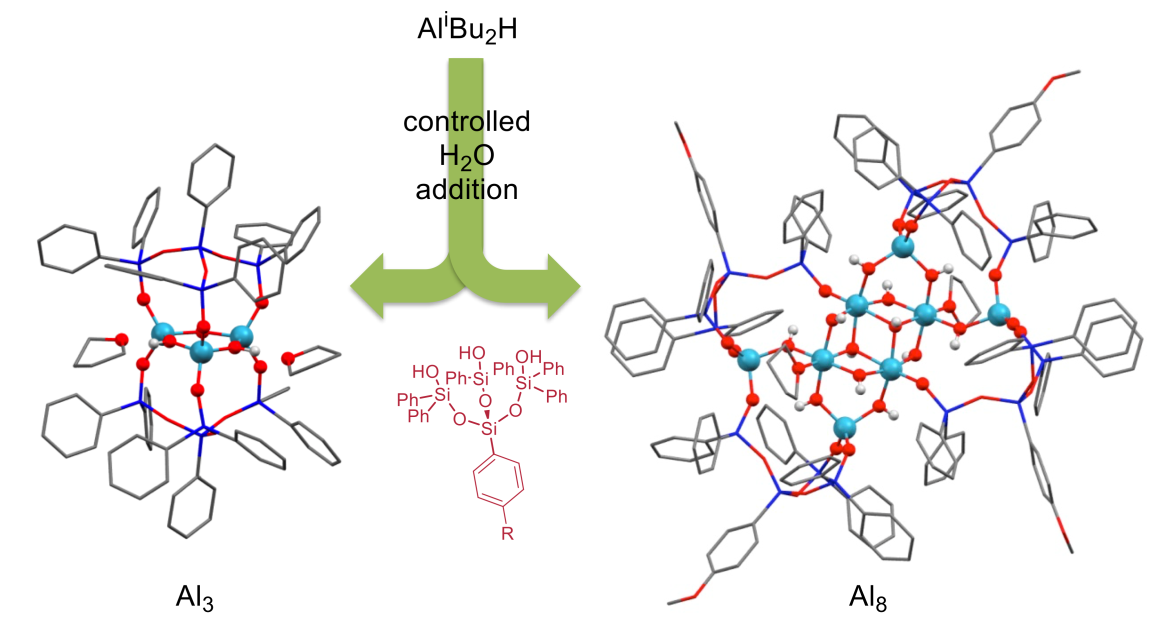
A third research area deals with molecular aluminosilicates. Here we are interested in the speciation and assembly of aluminium oxide/hydroxides setting out from mononuclear precursor compounds upon contact with water on their way to the respective solids. We are trapping species on this way by addition of suitable silanol ligand precursors, and in dependence on the amount of water added aluminium hydroxide clusters of different sizes can be isolated. Such clusters can be used as guides for interfacial reactions and hydrolytic processes for the solids, which cannot be investigated in a similar fashion. Finally, due to the solubility and stability of the clusters they can also be employed as Bronstedt acid catalysts in solution for reactions that are typically catalyzed by zeolites.
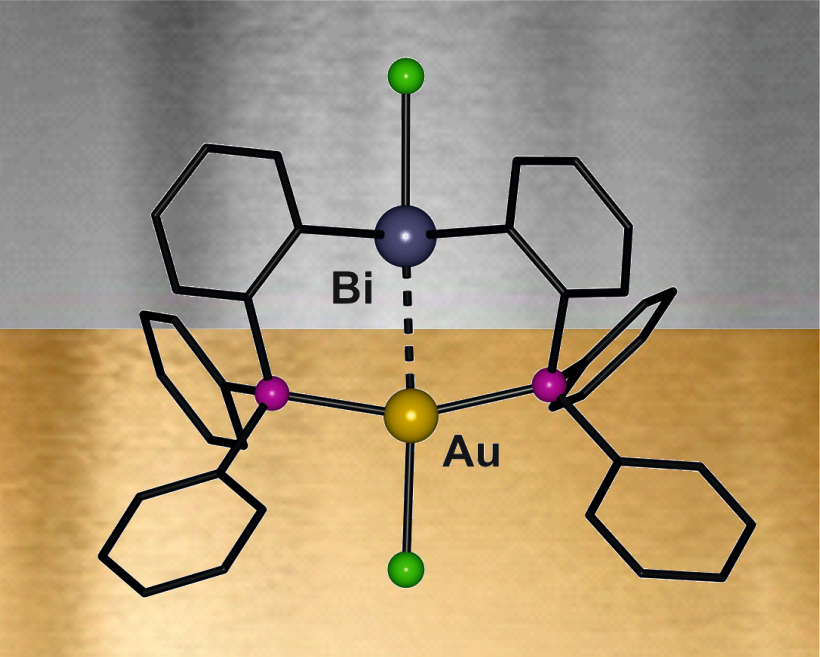
Compared to the other pnictogens bismuth – the heaviest of all stable elements – certainly has a unique character. Hence also its chemistry in the oxidation state +III frequently differs a lot from the one of its lighter homologues. This translates also into interesting properties of bismuth-containing materials, in particular of multimetallic systems. This in turn motivates the construction and investigation of molecular transition metal–bismuth compounds that (i) feature structural units of the materials but are soluble, (ii) that may be utilized as single source precursors (SSP), (iii) exhibit unknown types of bonding (new element combinations). We are thus investigating compounds with covalent Bi-M bonds as well as such with metallophilic interactions of the type Bi→M or Bi←M (M = transition metal).
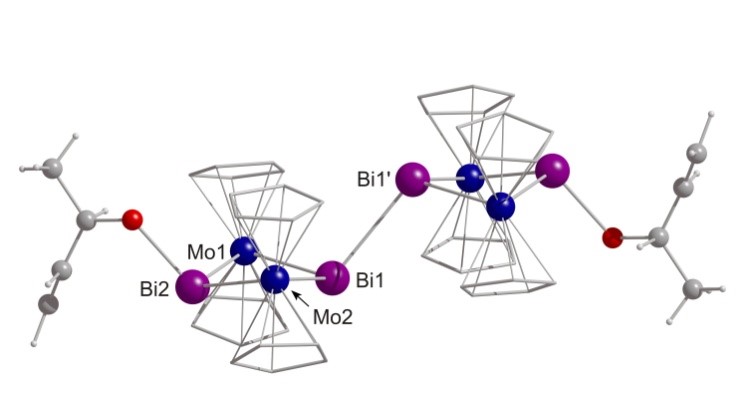
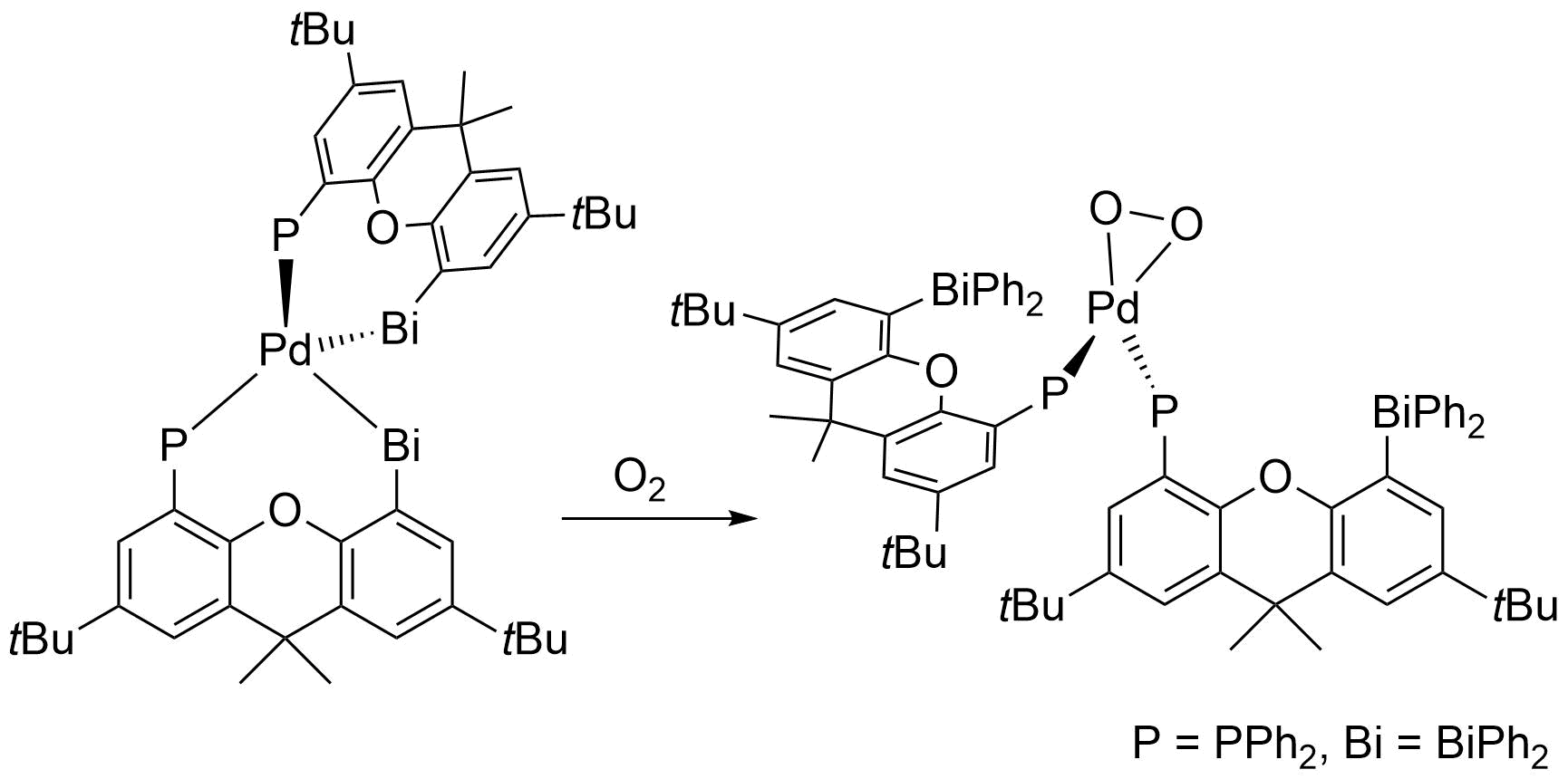